University of Minnesota researchers part of collaboration that casts doubt on 'sterile neutrino'
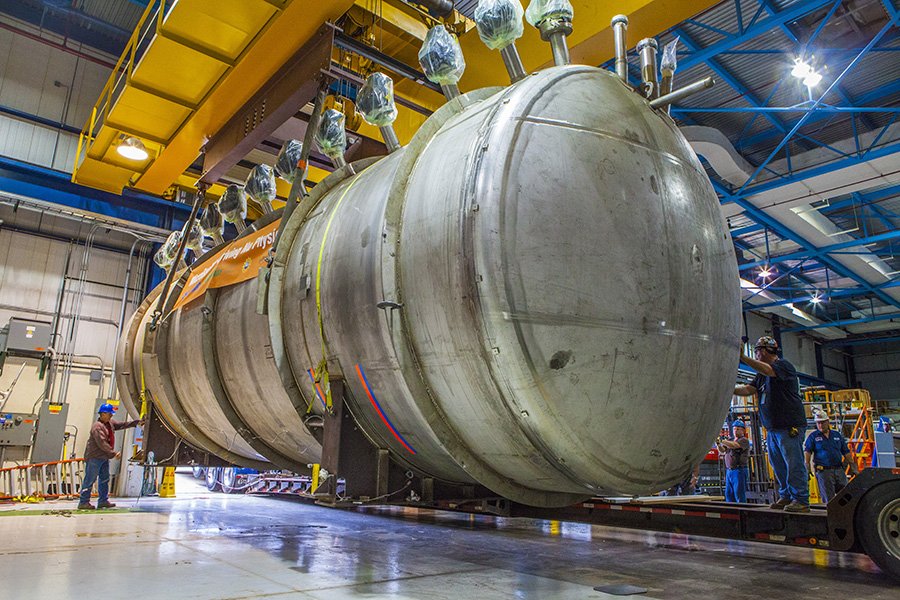
Experiment results provide new clues to the origins of matter and the Universe
MINNEAPOLIS / ST. PAUL (10/28/2021) — University of Minnesota Assistant Professor Andrew Furmanski and his team in the College of Science and Engineering’s School of Physics and Astronomy are part of an international collaboration of scientists who have possibly eliminated the theory related to the “sterile neutrino.”
Scientists believe that a better understanding of neutrinos, one of the most abundant and difficult-to-study particles, may lead to a clearer picture of the origins of matter and the inner workings of the Universe.
A neutrino is a subatomic particle that is very similar to an electron, but has no electrical charge and a very small mass, which might even be zero. Neutrinos are particularly intriguing to physicists, with a number of unanswered questions surrounding them. The Standard Model of Particle physics holds that there are three “flavors” of neutrino—the electron, muon and tau neutrino. Neutrinos can switch between these flavors in a particular way as they travel. This phenomenon is called “neutrino oscillation.” Scientists can use their knowledge of oscillations to predict how many neutrinos of any kind they expect to see when measuring them at various distances from their source.
The “sterile neutrino” hypothesis was proposed by particle theorists more than 20 years ago, as a possible explanation for anomalies and excesses seen in other neutrino experiments. Finding a new particle would be a major discovery and a radical shift in our understanding of the Universe.
However, four new complementary analyses released by the international Micro Booster Neutrino Experiment (MicroBooNE) collaboration all show the same thing—no sign of the sterile neutrino. Instead, the results align with the Standard Model of Particle Physics, scientists’ best theory of how the universe works. The data is consistent with what the Standard Model predicts: three kinds of neutrinos—no more, no less.
“After so many years of head scratching, we have managed to rule out several of the simplest explanations for the excess that MiniBooNE observed—that the excess could be caused by extra electron neutrinos, and that the excess could be caused by neutrinos producing photons more often than expected,” Furmanski said. “This still leaves us with many potential explanations for the MiniBooNE results, which we're going to be looking into over the coming years.”
MicroBooNE is the successor to an experiment called MinibooNE, both born out of the desire to investigate these anomalies further. MiniBooNE scientists also saw more particle events than calculations predicted. These strange neutrino beam results were followed by reports of missing electron neutrinos from radioactive sources and reactor neutrino experiments.
Sterile neutrinos emerged as a popular candidate to explain these odd results. While neutrinos are already tricky to detect, the proposed sterile neutrino would be even more elusive, responding only to the force of gravity. But because neutrinos flit between the different types, a sterile neutrino could impact the way neutrinos oscillate, leaving its signature in the data.
MicroBooNE is a neutrino detector roughly the size of a school bus that has operated since 2015. The detector is filled with 170 tons of pure liquid argon. Neutrinos bump into the dense, transparent liquid, releasing additional particles that the electronics can record. The resulting pictures show detailed particle paths and, crucially, distinguish electrons from photons.
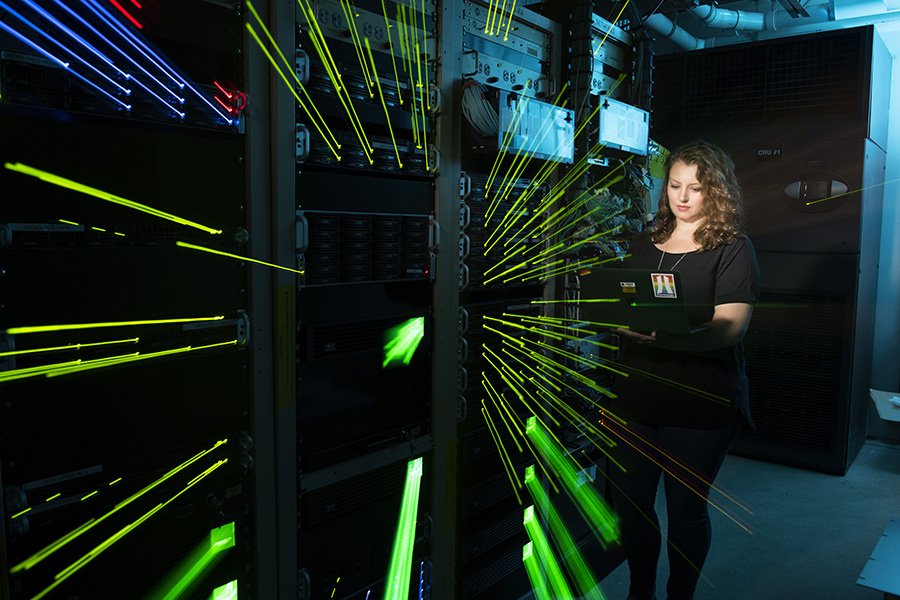
The international experiment has close to 200 collaborators from 36 institutions in five countries. They used cutting-edge technology to record spectacularly precise 3D images of neutrino events and examine particle interactions in detail—a much-needed probe into the subatomic world.
Furmanski has been a member of the MicroBooNE collaboration since he was a postdoctoral researcher in 2015. He was involved in installing and commissioning the neutrino detector. He also was responsible for operations for the first year of running. Since joining the faculty at the University of Minnesota, his team has had significant input on the experiment’s results, primarily related to improving the understanding of how neutrinos interact and how the detector works.
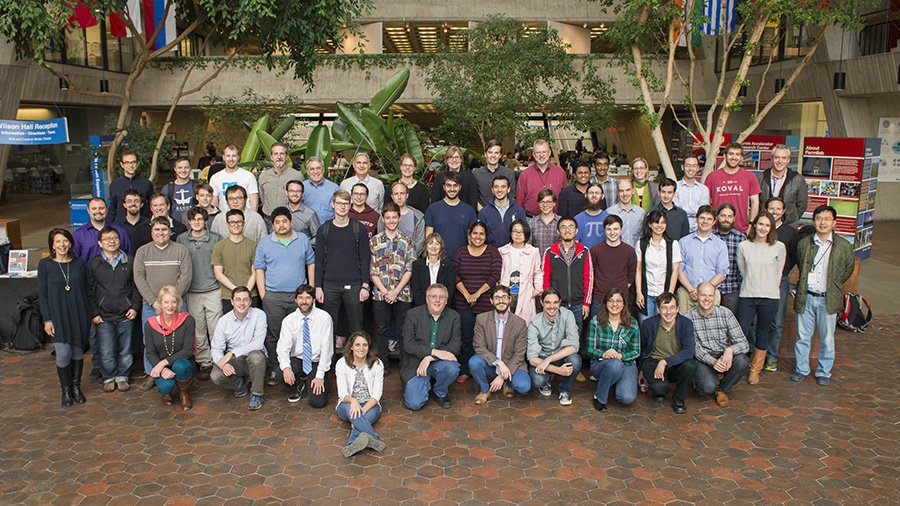
“Many of the limitations of the previous MiniBooNE detector are overcome using the new liquid argon technology in MicroBooNE, but the detector is complex and can be challenging to understand,” Furmanski said. “Our team developed ways of measuring the detector response to various particles and using these measurements to update how we simulate the detector.”
Another limitation in the MiniBooNE results was the modelling of neutrino interactions. Neutrinos interactions are rare so it can be difficult to measure enough of them to understand how they actually interact in detectors.
“MiniBooNE actually made huge progress in developing our understanding of neutrino interactions, but in the years since we have been able to develop new and improved models for neutrino interactions based on data from experiments around the world,” Furmanski said. “The team at the University of Minnesota was key to getting these models into our data analysis chain to make sure we aren't misinterpreting what we see.”
The team at the University of Minnesota is also involved in preparing for the addition of two other liquid argon detectors in the same beam as MicroBooNE, so they will have even more ways of explaining the MiniBooNE results. The upcoming DUNE experiment, a massive kiloton scale detector planned to be built in a mine in Lead, SD, is also going to use the same liquid argon technology, and these results from MicroBooNE demonstrate the power of this technology.
“DUNE is still some way off, but we are in the process of prototyping the detector components, which involves taking everything we learned from the MicroBooNE detector to improve this technology,” Furmanski added.
In addition to Furmanski, the University of Minnesota researchers involved in MicroBooNE include Chris Hilgenberg, a postdoctoral researcher, and graduate student Richie Diurba.
MicroBooNE is supported by the U.S. Department of Energy, U.S. National Science Foundation, Swiss National Science Foundation, U.K. Science and Technology Facilities Council, U.K. Royal Society, and European Union’s Horizon 2020.
Read Fermilab’s full news release.
For more information, visit the MicroBooNE website or take a virtual tour inside the MicroBooNE detector.