Research
Many cells exist in dynamic mechanical environments, necessitating constant adaptation to maintain tissue integrity. Biomechanical models can provide mechanistic insight into these adaptive processes and, if robust enough, could assist in physicians' treatment decisions. Our lab focuses on developing in vitro experimental methods and computational tools necessary to advance our understanding of cell-scale and tissue-scale mechanobiology and develop clinically-applicable mathematical models.
Neurotrauma

Chronic traumatic encephalopathy (CTE) is a neurodegenerative disease associated with repeated mild traumatic brain injury (TBI). CTE is among the many neurodegenerative diseases characterized as tauopathies, wherein the protein tau, which is usually associated with microtubules in the axons of neurons, becomes separated from microtubules, initiating a degenerative cascade and leading to eventual neurofunctional loss. There are currently no pharmacological treatments available for CTE patients, so any treatment that could limit or reverse tau-associated dysfunction would have an important impact on TBI patient outcomes.
We are designing a new stretchable multi-well plate for neuronal cell culture and a new high strain rate stretcher that can apply trauma-like loads to the cells in the plate. We will employ machine learning based algorithms to quickly and efficiently analyze the data collected from our new device. We plan to use the device to test inhibitors known to be effective against other aspects of neuronal injury. We also hope to determine biophysical mechanisms that drive tau mislocalization based on cell structure and orientation.
Cerebral Aneurysms
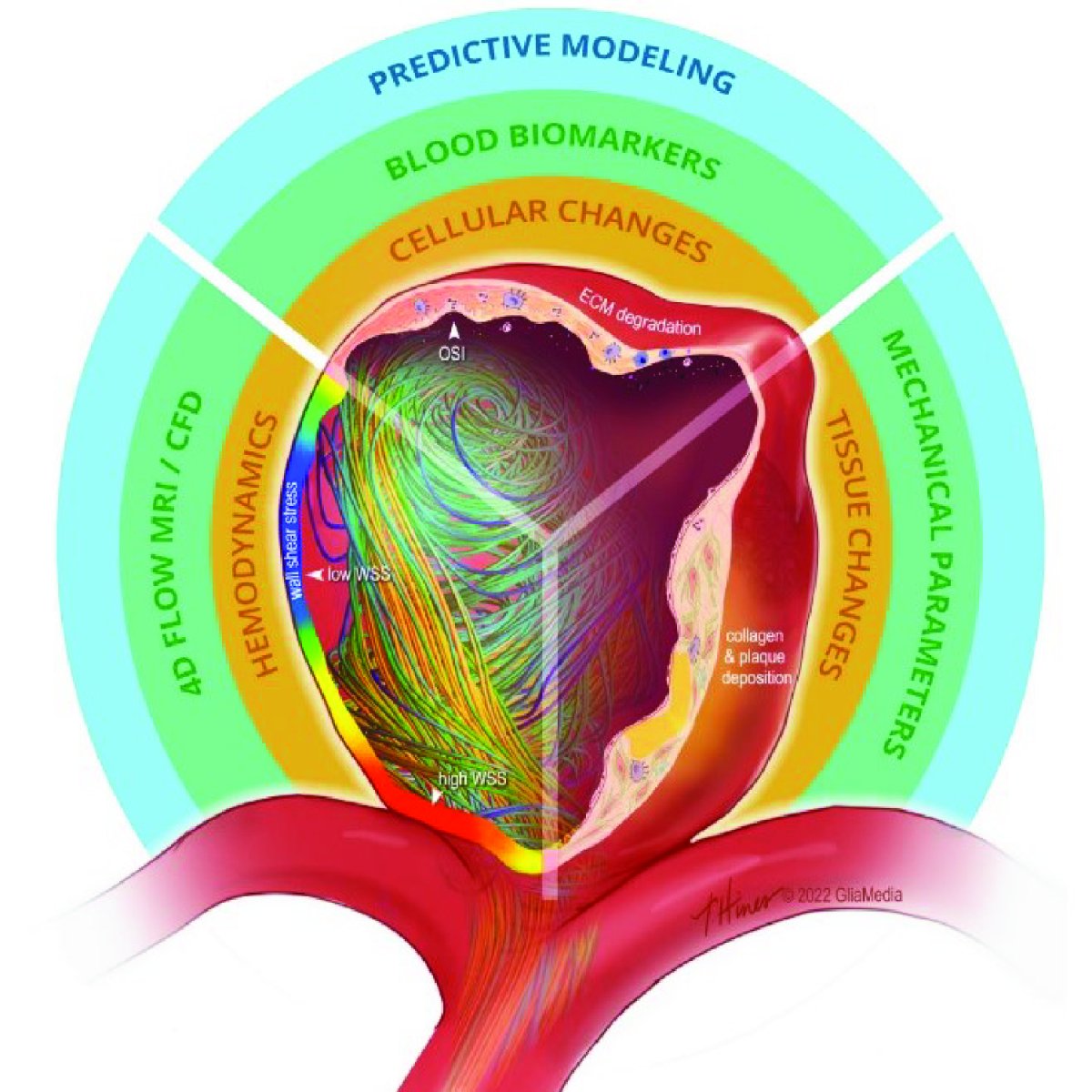
Cerebral aneurysms are out-pouching dilations of cerebral arteries caused by local wall weakening and maladaptive remodeling. Though rupture is relatively rare, the post-rupture survival rate is low, due to complications such as vasospasm and stroke. Since the majority of cerebral aneurysms are stable, the ability to predict rupture would both allow early intervention and eliminate unnecessary surgical procedures for stable aneurysms.
Using freshly excised human aneurysm tissue, we measure regional tissue-scale mechanical properties, ECM structure and composition, cell organization, and the rupture stress of cerebral aneurysms. We also develop and use computational models to elucidate the biophysical mechanisms that connect tissue properties to aneurysm rupture. We plan to use computational analyses of the architecture and blood flow mechanics within the aneurysm to connect these clinically-measurable metrics to clinically non-measurable material properties.
Cardiomyocyte Mechanics

There is a lack of fundamental understanding of how cardiac cells respond to complex mechanical loads, which drastically limits the innovation of intervention in disease settings. In particular, elucidating the functional adaptation response of cardiac tissues would provide an opportunity to guide surgeries, 3D tissue engineered hearts or patches, and construction of in vitro heart disease models for testing of interventions.
Using our novel cellular microbiaxial stretching microscopy techniques, we seek to elucidate the acute and long-term mechano-adaptive response of cardiomyocytes exposed to complex loads.
Other Nonlinear Cellular Mechanics
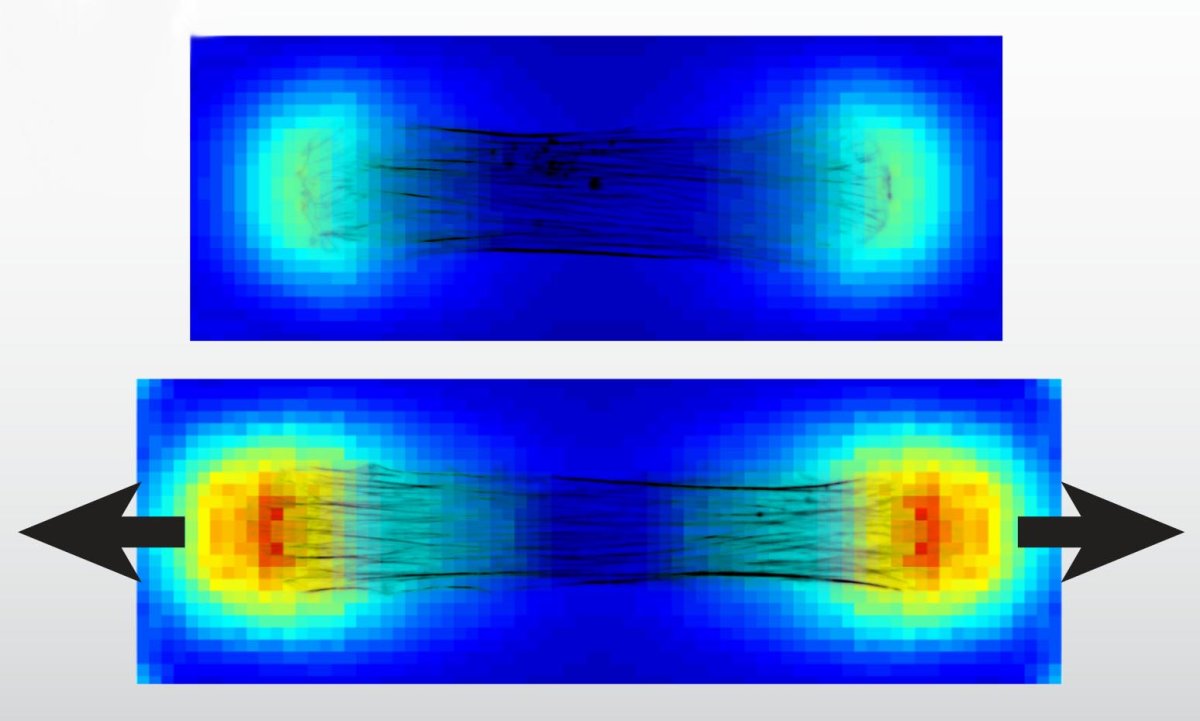
Most tissues are mechanically anisotropic and nonlinear at large strains. However, though cells within tissues have highly organized structures and undergo similar strains to the tissues they are a part of, cellular properties are normally reported as linear moduli. To understand how mechanical forces alter cellular behavior, it is necessary to describe the full material behavior of cells.
We are developing in vitro methods for characterizing aniostropic hyperelastic and viscoelastic constitutive behavior of cells, determining how those properties can be altered, and elucidating the role of adaptive property modulation in disease progression. Using what we learn from these characterizations, we can make predictions about growth and remodeling of blood vessels in genetic cardiovascular diseases, cerebrovascular/ neurodegenerative diseases, and in heart morphogenesis.