Seminars
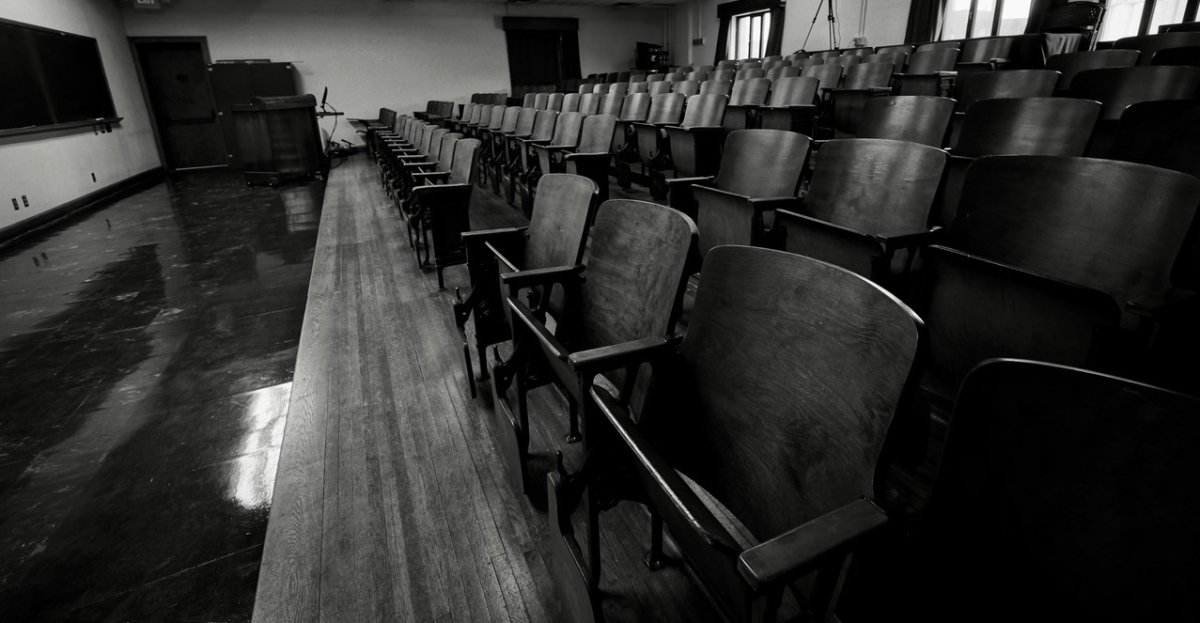
Every other week during the academic year, SAFL hosts prominent figures in environmental science and fluid mechanics. They come from all over the US and the world to share their insight and inspire us to tackle important questions in the field. These seminars are free and open to the public. Join us to learn about the latest research advancements and network with contacts in the field.
SAFL seminars are held on Tuesdays from 3:00 to 4:15 p.m. unless otherwise noted. Join us in the SAFL Auditorium or via Zoom.
Spring 2024 Seminar Series
Recordings
We will record seminars and post them here when given permission by the speaker. To see if a recording is available, scroll down this page to "Past Seminars."
Seminar Notifications
To sign-up for our SAFL Seminar email list, click here.
Related Seminar Series
Department of Civil, Environmental, and Geo- Engineering
Department of Earth Sciences
Department of Mechanical Engineering
Department of Aerospace Engineering and Mechanics
Center for Transportation Studies
Institute on the Environment
Water Resources Sciences
Minnesota Stormwater Seminar Series
Upcoming Seminars
There are no upcoming events matching your criteria.
Past Seminars
The Alvin Anderson Award Ceremony & Distinguished Lecture by Walt Musial
Tuesday, May 7, 2024, 3 p.m.
This is a hybrid event.
Attend in-person: St. Anthony Falls Laboratory, 2 Third Ave SE, Minneapolis, MN 55414
OR
Read About the Alvin Anderson Award
Distinguished Lecture:
Technical Challenges of Deploying Offshore Wind in U.S. Regions
Abstract: A massive transition is underway to convert the global energy supplies to net zero carbon emitting sources. Offshore wind is one of the most significant renewable energy sources available to the United States contributing to this transition. Nascent offshore wind systems are being deployed in Europe and in the Atlantic Ocean along the U.S. eastern seaboard but siting offshore wind energy projects in other regions will be necessary to meet our decarbonization goals. The south Atlantic, Gulf of Mexico, Great Lakes, and the Pacific Ocean each present new engineering challenges. To attain the necessary scale of deployment, new technology is needed to overcome design and deployment issues introduced by extreme hurricanes, surface ice floes, and deep water. The seminar will describe these issues and cover the state of the current research that is underway to overcome these challenges.
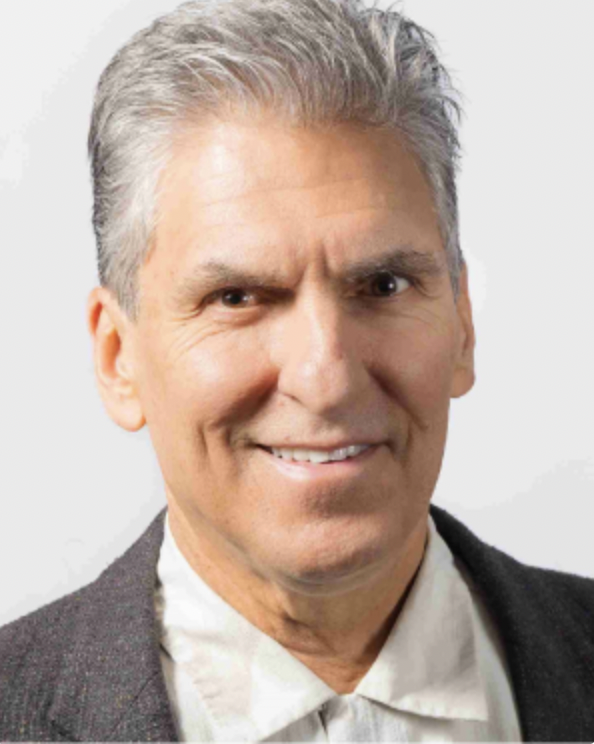
About the Distinguished Speaker: Walt Musial is the Chief Engineer for Offshore Wind at the National Renewable Energy Laboratory (NREL) where he has worked for 35 years. In 2003 he initiated the offshore wind energy research program at NREL which focuses on a wide range of industry needs and critical technology challenges. He founded and chairs the ACP Offshore Wind Standards Subcommittee and served for six years as the Senior Technical Advisor to the National Offshore Wind R&D Consortium. Through NREL’s strategic partnerships, Walt is leading multiple research activities to build the knowledge base for U.S. offshore wind regional frontiers, which include the Great Lakes, the Gulf of Mexico, the Gulf of Maine, and the Pacific Ocean. Previously, Walt developed and ran NREL’s full scale blade and drivetrain testing facilities for 15 years. Earlier, Walt worked five years as a field test engineer in the commercial wind energy industry in California. He studied Mechanical Engineering at the University of Massachusetts - Amherst, where he earned his bachelor’s and master’s degrees, specializing in energy conversion with a focus on wind energy engineering. He has over 120 publications and three patents.
Anderson Award Recipient: Mariel Jones, PhD candidate in the Water Resources Science (CFANS) program.
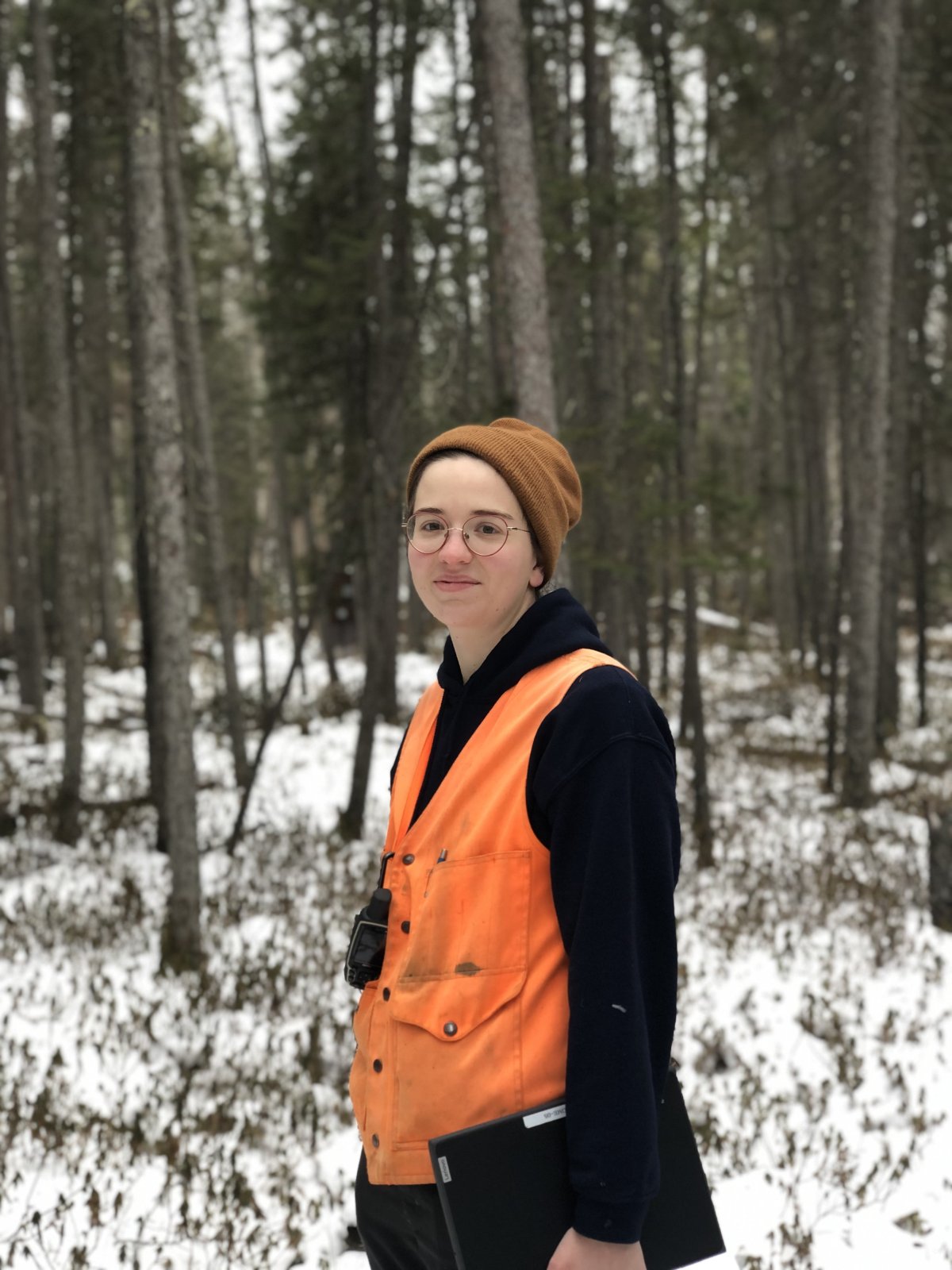
Abstract: Peatlands are an essential part of the global carbon cycle, storing 30% of the world’s total soil carbon while covering only 3% of the earth’s surface. Furthermore, peatland extent overlaps significantly with the area of receding permafrost—meaning more peatlands, and their carbon stores, will experience the effects of changing soil frost dynamics under current climate projections. While it is well known that soil frost can influence surface and subsurface flows, infiltration, and evapotranspiration, the when, where, and why these influences occur are still largely misunderstood, especially in watersheds with complex storage dynamics like peatlands. In this presentation, we provide a broad overview of three projects aimed at tackling this question of how, and under what conditions, frost affects the hydrological connectivity within peatland systems. First, using long term climatological data we show that soil frost influences the magnitude of annual streamflow rates and the timing of spring recharge during the winter season by controlling the cascade of water flow from the snowpack to the stream. In the second project, we invoke high spatial resolution field measurements to trace the influence of watershed architecture on snow accumulation and water storage within the watershed. Finally, we implement cutting edge hillslope-modelling techniques and field data assimilation to provide better predictions of water flow dynamics within the highly heterogeneous peatland-dominated watersheds and, by proxy, more accurate carbon dynamics in these global-scale models. Overall, this research aims to understand more about frost processes in peatland systems and understand the effects that continued increases in temperature and decreased water availability may have on peatland carbon dynamics.
About Marial Jones: Mariel Jones is a PhD candidate in the Water Resources Science (CFANS) program. Their work focuses on the nexus of climate, water, and landscape in peatland dominated watersheds. Currently, they are looking at the effects of climate change on snow regimes and increases in winter temperatures on soil frost and spring hydrological flows in Minnesota through both field and modelling lenses. Outside of research, Mariel is passionate about science communication and writes and illustrates environmental science comics. Before joining the Feng lab at the University of Minnesota and becoming immersed in ecohydrology, Mariel acquired a B.S. in Engineering Science and Mathematics & Statistics from Smith College in Massachusetts.
The Lorenz G. Straub Award and InterPore’s Kimberly-Clark Distinguished Lecture
Tuesday, April 23, 2024, 3 p.m.
This is a hybrid event.
Attend in-person: St. Anthony Falls Laboratory, 2 Third Ave SE, Minneapolis, MN 55414
OR
Read About the Lorenz G. Straub Award
The InterPore’s Kimberly-Clark Distinguished Lecture :
Fluids, Fingers, Fractures and Fractals: Patterns in Porous Media
Abstract: The displacement of one fluid by another in a porous medium gives rise to a rich variety of hydrodynamic instabilities. Beyond their scientific value as fascinating models of pattern formation, unstable porous-media flows are essential to understanding many natural and man-made processes, including water infiltration in the vadose zone, carbon dioxide injection and storage in deep saline aquifers, methane venting from organic-rich sediments, and fracturing from fluid injection. Here, we review a handful of these hydromechanical instabilities, elucidate the key physics at play, and point to modeling frameworks that permit quantitative assessments of their impact at the geologic scale.
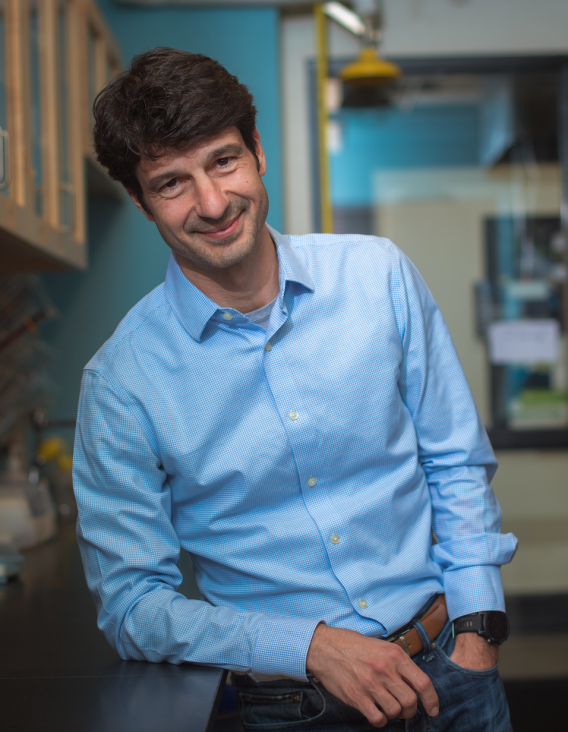
About the speaker: Ruben Juanes is professor in Civil and Environmental Engineering, and Earth, Atmospheric and Planetary Sciences at MIT, where he has been since 2006. He is an expert in fluid flow through porous media and in geomechanics, and has applied his research to the fields of energy resources, carbon capture and storage, gas hydrates, water infiltration and soil irrigation, and induced seismicity. He holds an undergraduate degree from University of A Coruña (Spain) and graduate degrees from UC Berkeley, all in Civil and Environmental Engineering. He is a fellow of the American Geophysical Union and is the 2024 InterPore Kimberly-Clark Distinguished Lecturer.
Straub Award Recipient: Dr. Rui Shi, University of Queensland, Adjunct Lecturer in the School of Civil Engineering
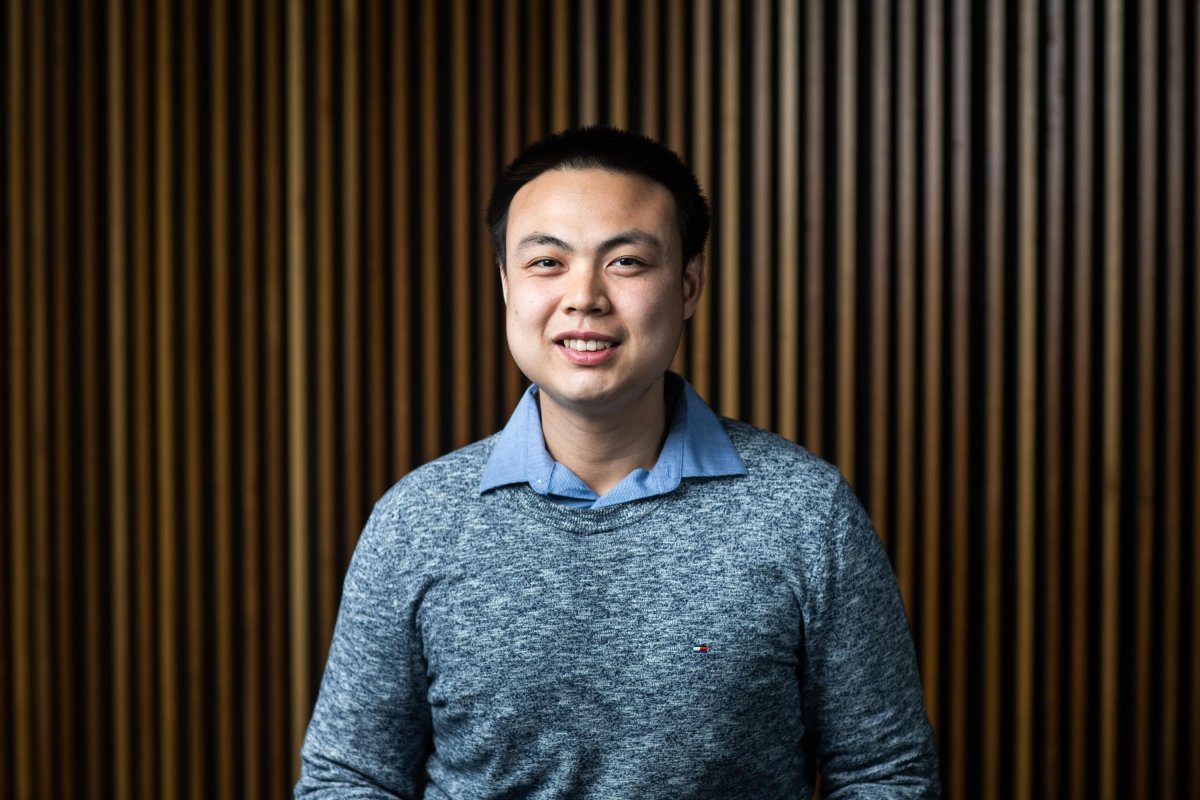
Abstract: Breaking bores, characterized with large-scale coherent structures, air entrainment and energetic free-surface motion, are commonly observed in nature, such as tidal rise in estuaries, dam-break type flows and coastal waves approaching inshore. The present study presents a novel experimental way to quantify the turbulent flow properties, particularly in aerated regions of the breaking bores. Numerically, the bores were simulated using a computational fluid dynamic (CFD) approach, solving both air and water phases for impossible Naiver-Stokes equations. The results of breaking bores were compared against other quasi-steady air-water flows, such as hydraulic jumps, spilling breakers and swash zone flows, suggesting a similar bubble-turbulence interaction process among these large-scale surface water breakers.
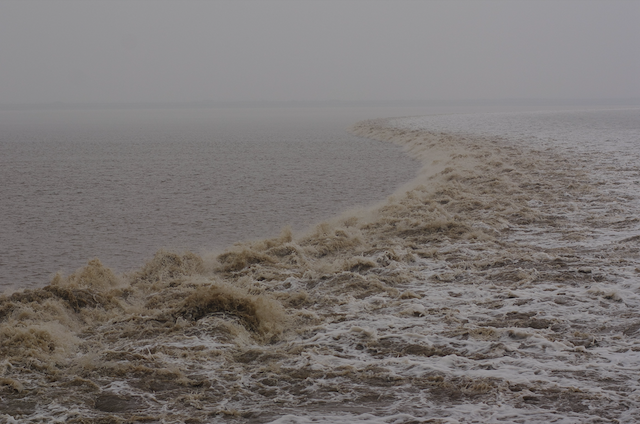
About Rui (Ray) Shi: Ray grew up in north-east China before moving to Australia for his bachelor's degree in civil engineering at the University of Queensland (UQ). During his bachelor studies, Ray developed a strong interest into fluid mechanics, leading to the completion of his PhD in coastal and hydraulic engineering under the supervision of Prof. Hubert Chanson. His PhD thesis was focused on experimental and numerical investigations on free-surface air-water flows (breaking bores, hydraulic jumps, self-aerated spillway flow, etc). At the late stage of the PhD program, Ray worked as a consulting water resource engineer with WSP Brisbane office, specializing in numerical flood modelling for infrastructure and mining projects. In 2022, Ray was employed as a hydrologist with Rio Tinto Iron Ore, responsible for technical supports regarding surface water challenges for open-cut mining operations.
The Heinz G. Stefan Fellowship & Distinguished Lecture by Dr. Sergio Fagherazzi
Tuesday, April 9, 2024, 3 p.m.
This is a hybrid event.
Attend in-person: St. Anthony Falls Laboratory, 2 Third Ave SE, Minneapolis, MN 55414
OR
Presentation of the Heinz G. Stefan Fellowship
Distinguished Speaker: Sergio Fagherazzi, Professor of geomorphology, hydrology, and coastal and marine geology in the Department of Earth and Environment at Boston University
Title of Lecture: Monitoring Coastal Wetlands Using Remote Sensing Data: the NASA Delta-X project
Abstract: The propagation of tides and riverine floodwater in coastal wetlands is controlled by subtle topographic differences and a thick vegetation canopy. High-resolution numerical models have been used in recent years to simulate fluxes across wetlands. However, these models are based on sparse field data that can lead to unreliable results. Here, we utilize high spatial-resolution, rapid repeat interferometric data from the Uninhabited Aerial Vehicle Synthetic Aperture Radar (UAVSAR) to provide a synoptic measurement of sub-canopy water-level change resulting from tide propagation into wetlands. These data are used to constrain crucial model parameters and improve the performance and realism of simulations of the Wax Lake wetlands in coastal Louisiana (USA). A sensitivity analysis shows that the boundary condition of river discharge should be calibrated first, followed by iterative correction of terrain elevation specified originally by a Digital Terrain Model derived from LiDAR measurements. The calibration of bed friction becomes important only with the boundary and topography calibrated. With the model parameters calibrated, the overall Nash-Sutcliffe model efficiency for water-level change increases from 0.15 to 0.53 with the RMSE reduced by 26%. In areas with dense wetland grasses, the LiDAR signal is unable to reach the soil surface, but the L-band UAVSAR instrument detects changes in water levels that can be used to infer the true ground elevation. The high spatial resolution and repeat-acquisition frequency (minutes to hours) observations provided by UAVSAR represent a groundbreaking opportunity for a deeper understanding of the complex hydrodynamics of coastal wetlands.
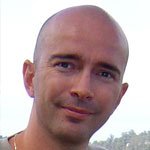
About the speaker: Sergio Fagherazzi studies geomorphology, hydrology, and coastal and marine geology. His research is oriented in three main directions: the morphological modeling of the continental shelf—formation and evolution of riverine networks during sea-level lowstands and subsequent channel filling during high-stands; the study of the hydrodynamics and morphology of salt marshes—observation, understanding, and modeling of creeks and channels developing on a salt marsh surface; and the numerical study of equations characteristic of coastal processes and hydrology—a dynamic model linking phenomena occurring at different spatial scales
Award Recipient: Guanju (William) Wei, Ph.D. student in Civil, Environmental, and Geo- Engineering
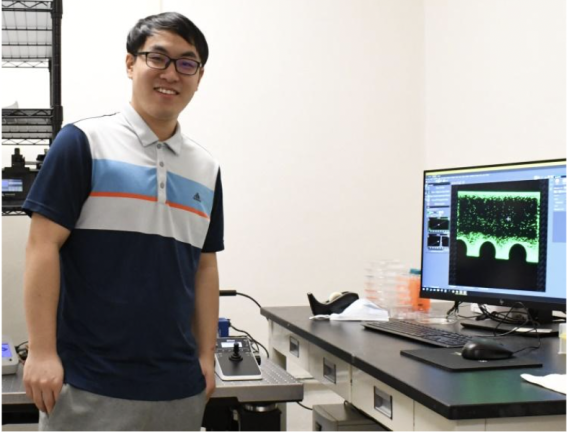
Talk Title: Microfluidic Investigation of the Biofilm Growth under Dynamic Fluid Environments
Abstract: Biofilms are consortiums of bacterial cells stick together by extracellular polymeric substances (EPSs). Biofilms can increase pathogenic contamination of drinking water, cause biofilm-related diseases, and alter the rate of sediment erosion in rivers and coasts. Meanwhile, some biofilms have been used for degrading polycyclic aromatic hydrocarbons (PAHs), enhancing oil recovery efficiency, and removing excess nutrients and contaminants from wastewater. Fundamental understandings of physical factors that control biofilm formation are needed yet currently lacking. Our goal is to systematically study the interactions between biofilm, flow, and particle transport, focusing on the effects of flow shear stress, different flow patterns, and surface properties on the biofilm formation process. We use microfluidic experiments, confocal imaging, numerical simulations, and mathematical modeling to study the interactions between biofilm, flow, and sediment transport. We developed imaging analysis methods using Image-J and Matlab to calculate the biofilm thickness, biofilm shape, and biofilm structure in an effort to demonstrate the effects of flows on biofilm growth. We are also interested in the biofilm's ability to bind sediment and micro-plastic particles and its effects on particle transport. We will create equations to predict the formation of biofilms under varying flow conditions. The results can be used in industrial, medical, and scientific fields to control biofilm growth.
About the recipient: I am currently a Ph.D. student in Dr. Judy Yang’s research laboratory. My academic journey began in China, where I earned both my bachelor's and master's degrees. My passion for science was ignited during my undergraduate years and has since been fueled by a deep curiosity to understand the nature of the world. In my present research, I combine microfluidic experiments and numerical simulations to study the interplay between fluid dynamics and bacterial behavior, as well as particle transport. I am especially interested in how different shear stress affects biofilm growth and, conversely, how biofilm growth impacts particle transport. Beyond the lab, I love watching sports like basketball, football, hokey, soccer, and more. In addition to sports, I enjoy playing video games, watching movies, cultivating plants, and caring for fish and turtles. What I appreciate most about our lab is the strong support and the valuable learning opportunities. I learn different skills from each lab member. Furthermore, being part of the Saint Anthony Falls Laboratory (SAFL) is an exciting experience. SAFL's multidisciplinary environment allows me to conduct a wide range of fascinating experiments.
The Charles C.S. Song Fellowship Award Ceremony & Distinguished Lecture by Dr. Michael Shelley
Tuesday, March 26, 2024, 3 p.m.
This is a hybrid event.
Attend in-person: St. Anthony Falls Laboratory, 2 Third Ave SE, Minneapolis, MN 55414
OR
Presentation of the Charles C.S. Song Fellowship Award
Distinguished Speaker: Dr. Michael Shelley, co-founder and co-director of the Courant Institute's Applied Mathematics Lab at New York University, and is also the Director of the Center for Computational Biology at the Flatiron Institute
Title of Lecture: Modeling self-organization in active fluids and materials.
Abstract: Biologically active materials -- like suspensions of swimming bacteria or other assemblies built of biological components -- can show strange, activity driven instabilities, organized states, and complex dynamics of a sort not found in more standard "nonliving" systems. We'll explore some of these phenomena through the lens of building, analyzing, and simulating models of prototype examples. These models can take the form of continuum models for the dynamics of suspensions of active particles (like bacteria), which can be analyzed for their dynamical bifurcation and nonlinear dynamics, or specialized software built to simulate very large systems of interacting active particles, and used to study swimming suspensions, growth and pattern formation in bacterial colonies, and cytoskeletal assemblies driven by molecular motors.
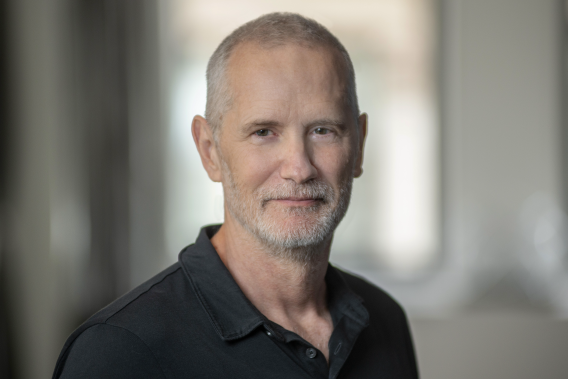
About: Dr. Michael J. Shelley is an applied mathematician who works on the modeling and simulation of complex systems arising in physics and biology. This has included free-boundary problems in fluids and materials science, singularity formation in partial differential equations, modeling visual perception in the primary visual cortex, dynamics of complex and active fluids, cellular biophysics, and fluid-structure interaction problems such as the flapping of flags, stream-lining in nature, and flapping flight. He is the co-founder and co-director of the Courant Institute's Applied Mathematics Lab at New York University, and is also the Director of the Center for Computational Biology at the Flatiron Institute. He holds a B.A. in mathematics from the University of Colorado and a Ph.D. in applied mathematics from the University of Arizona. He was a postdoctoral researcher at Princeton University and a member of the mathematics faculty at the University of Chicago before joining NYU. Shelley has received the François Frenkiel Award from the American Physical Society and the Julian Cole Lectureship from the Society for Industrial and Applied Mathematics, and he is a Fellow of both societies. He is also a Fellow of the American Academy of Arts and Sciences and a member of the National Academy of Sciences.
Award Recipient: Zih-Yin Chen is a PhD candidate in Mechanical Engineering at University of Minnesota
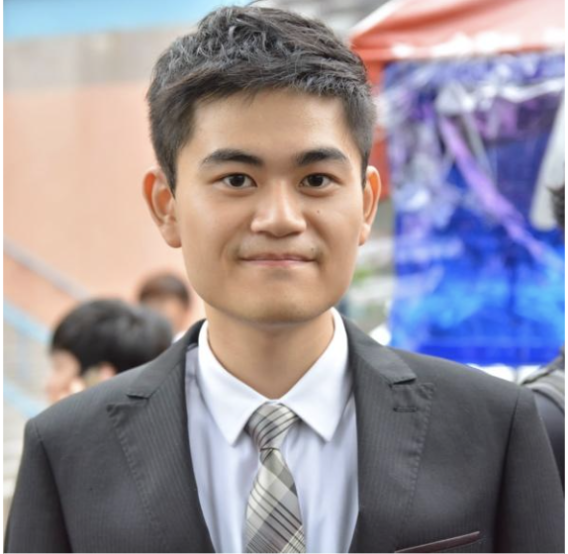
Talk Title: Thin film flow with an undulating surface
Abstract: Apple snails (Pomacea canaliculata) collect food particles on the free surface by deforming their feet and generating periodic undulations underneath the air-water interface. Inspired by this peculiar feeding behavior, we develop a 2D thin-film mathematical model to explore the physical mechanism of free surface flows driven by periodic undulations. We find the surface undulations cause normal stresses that deform the air-fluid interface and drive a net flow, which is directly controlled by the fluid properties and the undulation wave speed. Our model also demonstrates the effects of inertia that can significantly alter thin-film flows, in good agreement with the experimental observations. In this talk, we will discuss some preliminary model results along with the experimental findings and offer plausible physical mechanisms driving the flow system.
About the recipient: I am a PhD candidate in Mechanical Engineering and my advisor is Professor Sungyon Lee. My Ph.D. research aims to bring fundamental understanding of fluid dynamics processes at the fluid-fluid interface that are inspired by liquid coating applications, especially on the topics related to droplets and thin film dynamics. I use the theoretical modeling and the resultant simulations to rationalize the experimental findings and uncover the underlying physics. I received my BS and MS degree from National Taiwan University.
Nels Nelson Award Ceremony & Distinguished Lecture by Jennifer Stucker
Tuesday, March 12, 2024, 3 p.m.
This is a hybrid event.
Attend in-person: St. Anthony Falls Laboratory, 2 Third Ave SE, Minneapolis, MN 55414
OR
Presentation of the Nels Nelson Memorial Fellowship Award
Speaker: Jennifer Stucker, Senior Biologist, Offshore Wind Lead at Western EcoSystems Technology, Inc.
Title: A Multi-sensor Approach For Measuring Bird And Bat Collisions With Offshore Wind Turbines, and Other Insights from Collaborations with Engineers
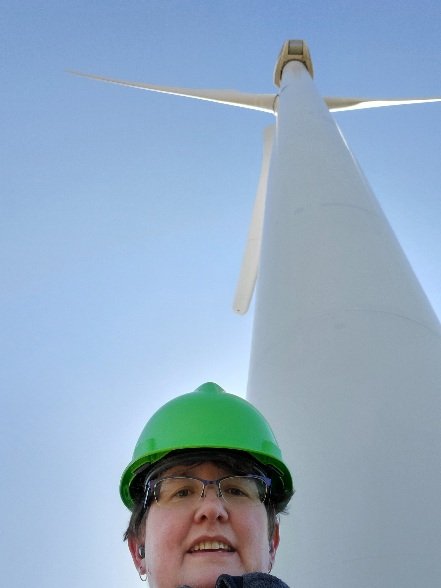
Abstract: Offshore wind energy development is poised for rapid growth and expansion worldwide to meet green-energy targets. Understanding the potential magnitude of bird and bat collisions at offshore wind facilities is important, because standardized carcass searches are impossible, and model-based estimates unvalidated. Collision monitoring systems can provide specific details about collisions, which are necessary to advance minimization strategies to reduce wind farm fatalities. Automated collision monitoring technologies can help inform and focus mitigation where needed. WEST leads a collaboration with the Netherlands Organisation for Applied Scientific Research and the National Renewable Energy Laboratory (NREL) to advance the validation of the WT-Bird® system for detecting and quantifying bird and bat collisions at offshore wind turbines. The effort is funded primarily by the US DOE's Wind Energy Technologies Office. The objective is to advance the WT-Bird® system to detect large, medium, and small bird and bat collisions during day and nighttime hours. Three major technological advancements from the project are to: 1) improve the sensors to detect collisions of small birds and bats, 2) integrate machine learning algorithms to process imagery data collected by the cameras, and 3) create a launching system to realistically simulate collisions. The updated system is designed to detect collisions including objects as small as 8 grams using acceleration sensors installed inside of the turbine blades. Independently operating cameras installed at the base of the turbine document bird and bat presence near the camera, and document collision events. The system was tested at a single turbine at NREL’s campus using the projectile launching system, where the detection rate exceeded 70% across all size classes. Subsequently, the system was installed on the University of Minnesota turbine for trials with a WEST edge-AI system processing camera imagery to identify birds and bats within the camera view, and validated with independent ground-based fatality searches. The estimate from the WTBird® detections (18.46 fatalities) was within the 90% confidence interval of the ground-based estimate (16.88) with a difference in the point estimates of only 1.58 fatalities.
Jennifer will talk about how biologists and engineers can and should work together to tackle wildlife and energy challenges to support the blue and green economies. She will provide key insights on talking a common language.
About: Jennifer Stucker, PhD is a Senior Biologist and Offshore Wind Lead with Western EcoSystems Technology, Inc, an environmental and statistical consulting firm. She has served as the project manager and biologist for this US Department of Energy funded project, A Multi-sensor Approach for Measuring Bird and Bat Collisions with Offshore Wind Turbines. Dr. Stucker received her BA from Wittenberg University in Ohio, before completing two degrees at the University of Minnesota, a MS in Wildlife Conservation, and a Ph.D. in Conservation Biology. Prior to working with WEST, she worked for USGS as a Research Wildlife Biologist for 10 years. Over the last 18 years, Jennifer has had experience designing effectiveness and monitoring studies for wildlife interactions with hydropower, wind, and solar facilities during planning and operations. When not working, you will find her with her family, and training dogs.
Award Recipient: Xiating Chen, PhD Candidate in Water Resources Engineering at the Saint Anthony Falls Laboratory
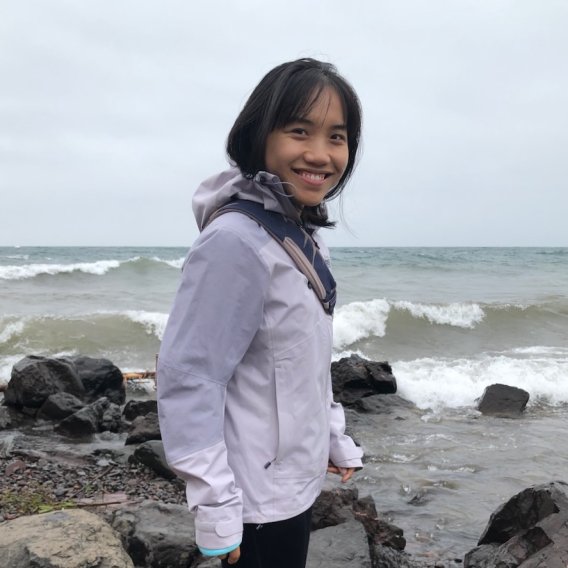
Abstract: TBD
About the recipient: Xiating Chen is a PhD candidate in water resources engineering at the St. Anthony Falls Laboratory studying under Professor Xue Feng. Her research is focused on eco-hydrological functions of urban trees and other green infrastructure at both local and watershed-scale, through combined field observations and modeling approaches. Xiating received her Bachelor of Science in Engineering degree from Duke University.
Edward Silberman Award Ceremony & Distinguished Lecture by Neal Iverson
Tuesday, Feb. 20, 2024, 3 p.m.
This is a hybrid event.
Attend in-person: St. Anthony Falls Laboratory, 2 Third Ave SE, Minneapolis, MN 55414
OR
Presentation of the 2023 Edward Silberman Fellowship Award
Speaker: Neal Iverson, Distinguished Professor in the Department of Geological and Atmospheric Sciences at Iowa State University
Title: A universal slip law for glaciers?
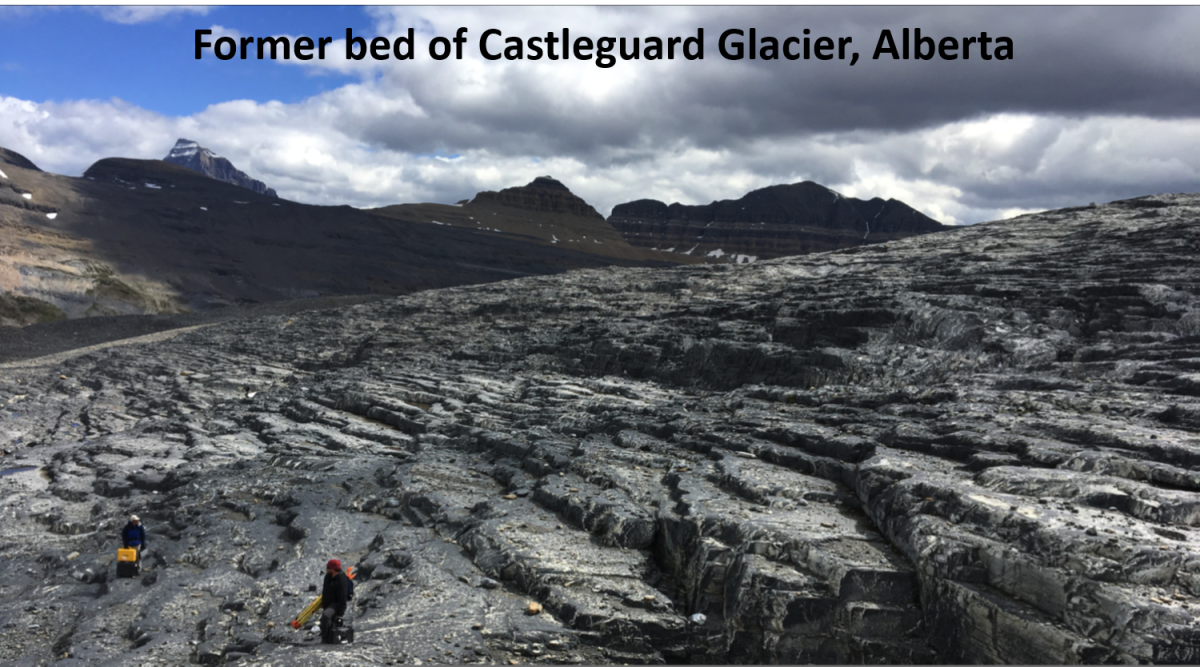
Abstract: Ice discharge to the oceans from ice sheets and associated sea-level rise result largely from rapid slip of marine-terminating ice streams over their beds. Slip occurs over either rough bedrock (“hard” bed) or deformable till (“soft” bed). For each bed type, glacier flow models require a constitutive law that describes the relationship between shear stress and slip velocity. Experimental studies of ice slip over a soft bed and results of a three-dimensional numerical model of glacier slip applied to measured hard-bed topography point to a slip law that has a common form for the two bed types. This finding has the potential to simplify and improve estimations of glacier discharges to the oceans. Regardless of bed type, the sensitivity of slip velocity to increasing stress on glacier beds—caused for example by the melting of buttressing ice shelves in the warming oceans—is larger than currently assumed in most ice sheet models.
About: Neal Iverson is a Distinguished Professor in the Department of Geological and Atmospheric Sciences at Iowa State University. He received his Ph.D. from the University of Minnesota in 1989. His research focuses on glacier flow, glacial erosion and sedimentation, and genesis of glacial landforms. He is a recipient of the Geological Society of America’s Kirk Bryan Award and Arthur L. Day Medal.
Award Recipient: Jiaqi Li, PhD Candidate in Mechanical Engineering at the Saint Anthony Falls Laboratory
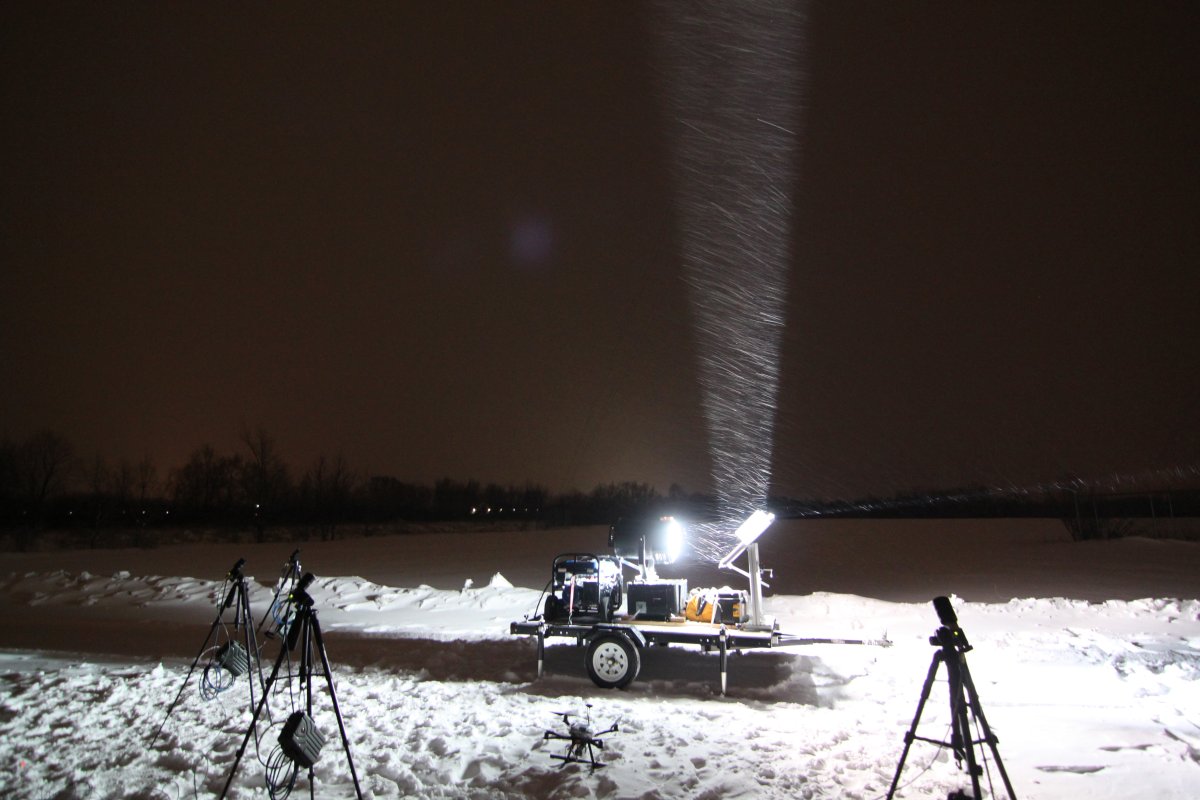
Abstract: Fluid flows, especially turbulent flows, are ubiquitous in the environment and critical for our daily life across various sectors, including healthcare and manufacturing. Our research focuses on a set of techniques to explore the dynamics of fluids and their interactions with particulates. At a larger scale, we conducted field experiments to measure the settling snow particles, using both planar and three-dimensional imaging techniques, alongside a snow particle analyzer. Our findings reveal preferential sweeping as a key mechanism in snow-turbulence interactions and demonstrate how snow morphology influences their fall behavior in low-turbulence environments, with implications for meteorological and climate predictions. On the smaller scales, we investigated the internal flow within evaporating sessile colloidal droplets. Through experimental observations and theoretical modeling, we elucidate the mechanisms governing particle deposition patterns after droplet evaporation, shedding lights on applications such as printing-based manufacturing and blood disease diagnostics. In addition, we have developed a holography-based technique for direct vorticity measurement. It has been applied to the study of elliptical vortex rings, revealing the vorticity fluctuations caused by their unsteady behaviors. This method has potential for directly measure the spatial and temporal vorticity variation of thin vortex tubes in turbulence, providing new insights into scale interactions and energy dissipation of these turbulent eddies.
About the recipient: Jiaqi Li is a PhD candidate in Mechanical Engineering at the St. Anthony Falls Laboratory of the University of Minnesota. He is currently working with Prof. Jiarong Hong on experimental fluid mechanics. His research focuses on understanding the interactions between turbulent flow and falling snow particles with various shapes for improving weather forecasting and climate modeling. He also worked extensively with digital inline holography, including developing a method for direct vorticity measurement and a snow particle analyzer for measuring snow size, shape, and density, as well as investigating internal flow and deposition patterns of sessile colloidal droplets. Before starting his PhD journey, Jiaqi received his bachelor’s degree from the University of Science and Technology of China in Energy and Power Engineering.
Permafrost thaw and methane release in the Arctic
Tuesday, Jan. 23, 2024, 3 p.m.
This is a hybrid event.
Attend in-person: St. Anthony Falls Laboratory, 2 Third Ave SE, Minneapolis, MN 55414
OR
Katey Walter Anthony is an Aquatic Ecologist and Professor at the University of Alaska Fairbanks
Abstract: Permafrost thaw beneath Arctic lakes and other thermokarst (thaw) features leads to large emissions of methane, a potent greenhouse gas to the atmosphere. In turn, methane contributes to global climate warming, which leads to more permafrost thaw in a positive feedback cycle. This talk will uncover natural sources of methane in the Arctic, which come from both the organic matter decay activity of microoganisms in lake bottoms and from geologic sources deep within the Earth. This talk will also present mechanisms of carbon sequestration by the same permafrost thaw features, a natural process that mitigates greenhouse gas losses, and over the long term can lead to net climate cooling. Using field work and novel remote sensing methods, I will consider the important balance between positive and negative feedbacks in fate of permafrost thaw and climate change in the past (since the last deglaciation), present, and future (until year 2100).
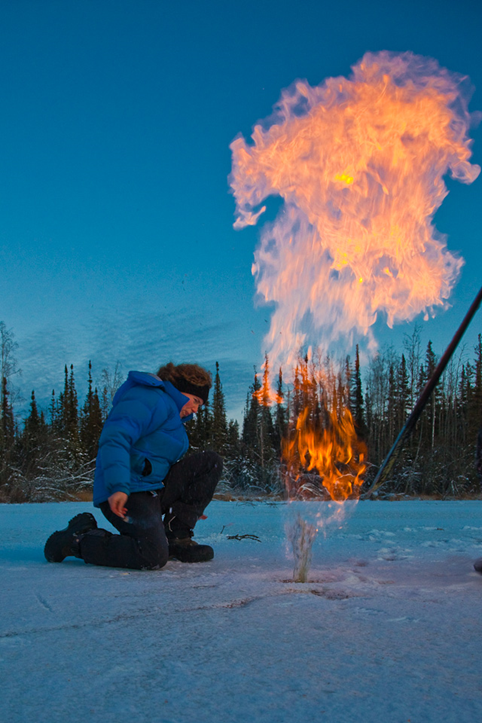
About: Katey Walter Anthony is an Aquatic Ecologist and Professor at the University of Alaska Fairbanks. Her research focuses on methane emissions from Arctic lakes, the degradation of permafrost, and its feedbacks to global climate processes through the carbon cycle. She has over 25 years’ experience conducting field work in Alaska and Russia, is a science team member of the National Aeronautics and Space Administration Arctic-Boreal Vulnerability Experiment, and a member of the Permafrost Carbon Network. She received the National Wildlife Federation Award in 2009, National Geographic Society Early Explorer’s Award in 2009, Mount Holyoke College Mary Lyon Award in 2010, WINGS WorldQuest Award in 2011, and the University of Alaska Usibelli Distinguished Research Award in 2019. Anthony received a B.A. in geology from Mount Holyoke College, an M.S. in restoration ecology from the University of California, Davis, and a Ph.D. in aquatic biology from the University of Alaska Fairbanks. She previously served on the National Academies of Sciences, Engineering, and Medicine’s Polar Research Board.
Dynamics of large irregular particles in turbulent flow
Tuesday, Dec. 12, 2023, 3 p.m.
This is a hybrid event.
Attend in-person: St. Anthony Falls Laboratory, 2 Third Ave SE, Minneapolis, MN 55414
OR
Margaret Byron, Assistant Professor of Mechanical Engineering at Penn State
Abstract: Environmental flows are rarely single-phase: they often carry particles like dust, sediment, flocs, or even living organisms. The details of how these particles are transported can have significant impacts on engineering problems; we must therefore understand the physics behind their interactions with the surrounding flow. However, the particles we are interested in are frequently nonspherical, nontrivially large compared to the flow scales, and or otherwise irregular (e.g. made of multiple materials, or having a nonuniform mass distribution). Such particles are sometimes treated as point masses, or as semi-passive flow tracers—but this simplified view doesn’t capture the full variation of the particles’ behavior, especially in turbulence. In this talk, we will present results from recent laboratory experiments and explore how varying particle shape, size, and mass distribution affect their dynamics in environmental turbulence, and discuss implications for a wide range of engineering problems including sediment transport, microplastic pollution, and the global carbon cycle.
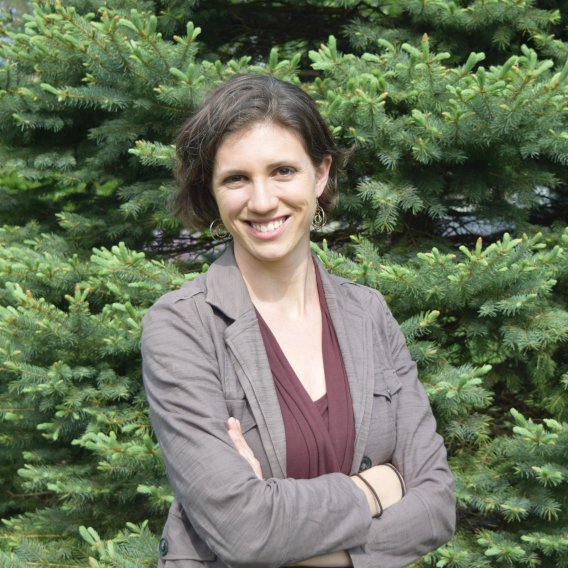
About: Margaret L. Byron is an Assistant Professor of Mechanical Engineering at Penn State University, where she directs the Environmental and Biological Fluid Mechanics (EBFM) Laboratory. She received her B.S.E. from Princeton University in Mechanical and Aerospace Engineering (2010), and her M.S. and Ph.D. from the University of California Berkeley (2012/2015). From 2015 – 2017 she was an NSF Postdoctoral Fellow in Biology at the University of California Irvine. She is a recipient of the American Chemical Society Doctoral New Investigator Award (2019), the Arnold and Mabel Beckman Foundation Young Investigator Award (2021), and the NSF CAREER Award (2022). Dr. Byron’s group studies the interactions between organisms and particles in environmental flows, with a particular focus on intermediate scales where inertial and viscous fluid forces are both important. She is interested in how animals control their position and orientation in turbulence, how swimming strategies scale with size and speed, and what this implies for their overall behavior and distribution in aquatic environments. She is also exploring the effects of particles’ size, shape, and mass properties on their kinematics in environmental flows; these problems have implications for sediment and pollutant transport.
Roger E.A. Arndt Fellowship Award Ceremony & Distinguished Lecture by Alfredo Soldati
Wednesday, Nov. 15, 2023, 11 a.m.
This is a hybrid event.
Attend in-person: St. Anthony Falls Laboratory, 2 Third Ave SE, Minneapolis, MN 55414
OR
Presentation of the 2023 Roger E.A. Arndt Fellowship
Distinguished Speaker: Alfredo Soldati, Professor of Fluid Mechanics at the Technische Universität Wien – TU Wien (Austria)
Title: Dynamics of complex particles in turbulence
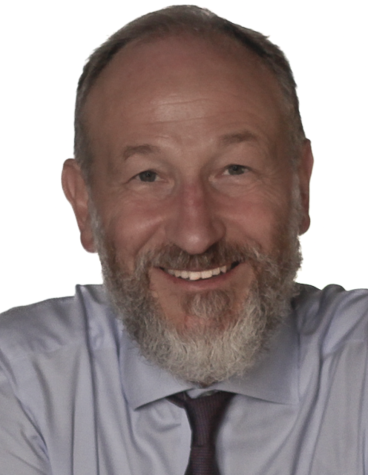
Abstract: Turbulent flows with suspended anisotropic particles of non-spherical shape are a common occurrence in many industrial (e.g. paper making, pharmaceutical processing, soot emission) and natural processes (e.g. pollen species, icy clouds, and plankton and marine snow). The dynamics of small anisotropic particles is determined by velocity gradients and in turn by the smallest turbulence scales, and although we have a fundamental understanding on the dynamics of such turbulence scales, since no closed form of the drag on non-spherical particles is available, predictive understanding of the dynamics of anisotropic particles remains elusive. This insufficient knowledge is at the basis of our lack of predictive tools to describe anthropogenic oceanic pollution of plastic microfibers, which are elongated, anisotropic and of the order of the smallest scales of turbulence. In this talk, we will briefly review the models used to describe the dynamics of small ellipsoidal particles in turbulence, and then we will add a further complexity consisting on allowing the elongated particles to be slightly curved: this slight curvature will be instrumental in determining the complete rotational dynamics of the particles in turbulence. Experiments performed in the TU Wien Turbulent Water Channel will be presented elaborating on the full dynamics of small non- axisymmetric –quasi-straight to slightly curved – fibers. We will highlight the role of fibers curvature on spinning and tumbling rates and we will propose conceptual models and scalings laws.
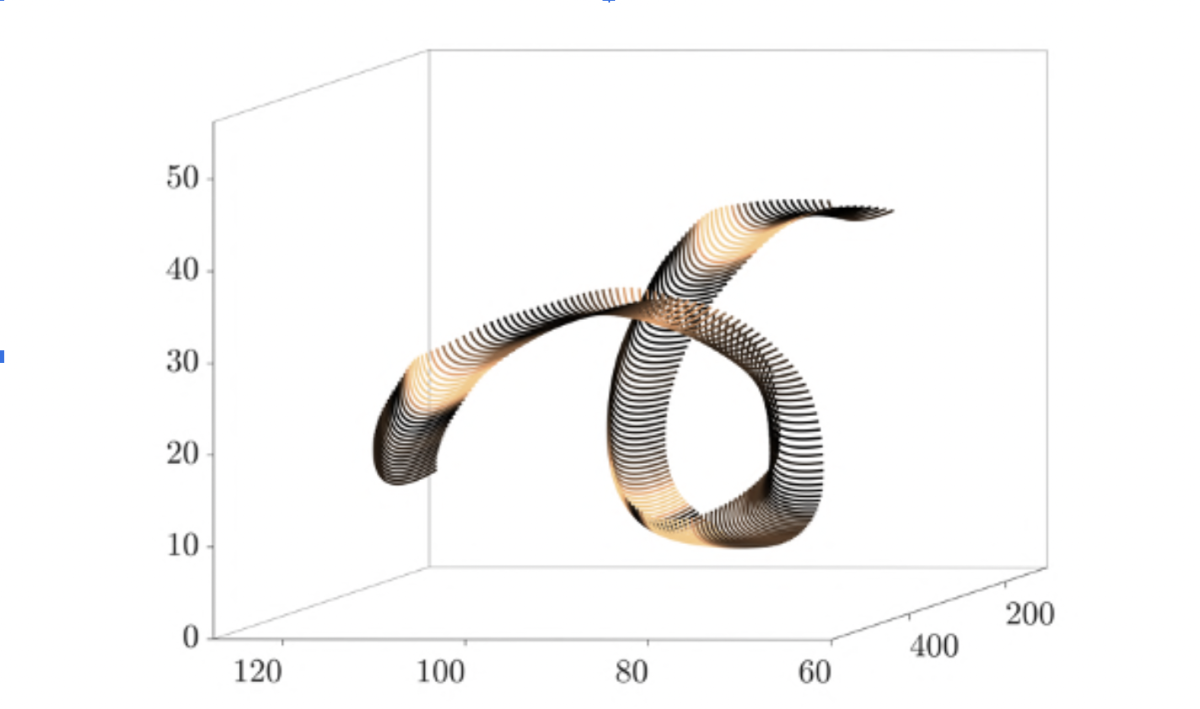
Figure 1: Measurement of a non-axisymmetric fibre traveling close to the wall of a turbulent channel flow, represented here by the grey surface. Front view is shown. Fibre is colored according to the instantaneous tumbling rate ΩtΩt normalized by the mean value computed over the entire track |ΩtΩt| (From M. Alipour, M. De Paoli, and A. Soldati (2022) “Influence of Reynolds number on the dynamics of long non-axisymmetric fibers in channel flow turbulence”, J. Fluid Mech. 934, A18-27.)
About: Alfredo Soldati is a Professor of Fluid Mechanics at the Technische Universität Wien – TU Wien (Austria). The focus of the research of his group is on multiphase flows, and specifically on the fundamentals of turbulent dispersed flows of particles, bubbles, and droplets.
After his PhD in chemical Engineering at the University of Pisa (Italy), and a visiting appointment at UCSB (CA), Dr. Soldati became assistant, associate and full professor at the University of Udine. He has been guest Professor at EPFL (Switzerland), at INP Toulouse (France) and at Scuola Superiore Sant’Anna Pisa (Italy). He is fellow of the American Physical Society (APS) and of the European Mechanics Society (EUROMECH), the recipient of the 2007 Knapp award and of the 2015 Lewis F. Moody Award from the American Society of Mechanical Engineers (ASME), the recipient of the International Prize and Gold Medal Panetti–Ferrari, from Accademia delle Scienze di Torino, Italia, and is awarded the 2020 ASME Freeman Scholarship and Lecture. Dr. Soldati is currently part time professor at University of Udine, and he is the Rector of the International Center for Mechanical Sciences, CISM. Dr. Soldati is Co-Editor in Chief of International Journal of Multiphase Flow.
Award Recipient: Amy Tinklenberg, Aerospace Engineering and Mechanics at the University of Minnesota.
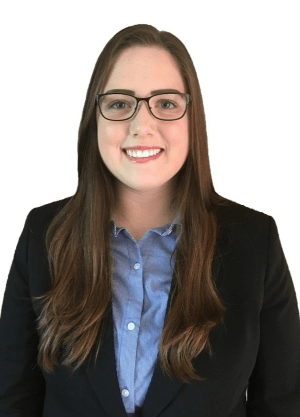
Abstract: Predicting the fall speed of frozen hydrometeors in the atmosphere is complicated by their unique geometries as well as by the air turbulence. Snowflakes and ice crystals often exhibit rimed branches resembling perforated shapes, which make them permeable to the air flow. This alters their wake dynamics in ways that have not been characterized in turbulence. As analogue to plate crystals, here we consider thin disks of 3 mm in diameter and compare geometries with and without perforations. These are dropped at controlled volume fractions into a large chamber generating a large region of approximately zero-mean-flow homogeneous air turbulence. Two turbulence levels are considered and contrasted with quiescent air conditions. The disks are imaged at 4300 Hz and their linear and rotational motion is reconstructed. Comparison of the solid and perforated disk behaviors in quiescent air shows that the perforated disks fall at a slower velocity than the solid disks. The perforated disks also fall more stably compared to the solid, which may either fall flat or tumble. In turbulence, both disk types experience a reduction in their average settling velocity, as compared to quiescent air, but the perforated disks are more mildly influenced by this effect.
About the recipient: Amy is a Ph.D. Candidate in Aerospace Engineering and Mechanics at the University of Minnesota. She works with advisor Filippo Coletti (ETH Zürich) and co-advisor Michele Guala (UMN). She experimentally investigates the settling dynamics of solid and perforated disks in both quiescent and turbulent air, aimed at better understanding and predicting frozen hydrometeor settling in the atmosphere. Originally from Minnesota, she received her Bachelor's in Mechanical Engineering from the University of St. Thomas (2019), followed by her Master’s in Aerospace Engineering and Mechanics from the University of Minnesota (2022).
The role of meltwater in reshaping the structures of icy porous media
Tuesday, Oct. 17, 2023, 3 p.m.
This is a hybrid event.
Attend in-person: St. Anthony Falls Laboratory, 2 Third Ave SE, Minneapolis, MN 55414
OR
Xiaojing (Ruby) Fu, Assistant Professor of Mechanical and Civil Engineering at California Institute of Technology.
Abstract: Icy porous materials such as snow or firn are ubiquitous in both Earth and planetary settings. Their microstructures (e.g., porosity) play an important role in dictating the reflectivity, fluid storage capacity, thermal conductivity, and mechanical properties of the larger-scale systems. Thus, understanding the complex physics that control the microstructure evolution of icy porous media is an important component in creating robust predictions of Earth’s cryosphere in response to climate warming, and in devising engineering strategies for the exploration of icy moons in our solar system. While it is well known that gravity-driven compaction leads to densification of the pore structure over depth, less is known about the role of meltwater in reshaping the structures of icy porous media across scales.
In this talk, I will describe our recent efforts in understanding how the movement of liquid water through porous ice reshapes its porosity structure via flow instability and phase transitions of water. I will first discuss the pore-scale problem and present a phase-field model that simultaneously captures the phase transitions of water amongst its liquid, solid and vapor phases. With this model, we show that the presence of quiescent meltwater films can accelerate the microstructural coarsening of porous snow during the process of metamorphism. Next, I will describe a Darcy-scale model that demonstrates how melt refreezing coupled with unstable infiltration reshapes the porosity structure of snow and leads to the formation of ice pipes and ice lenses commonly observed in the field. I will conclude by discussing the implications of these new physical insights for large-scale meltwater transport and hydrological processes in snow and glacial systems.
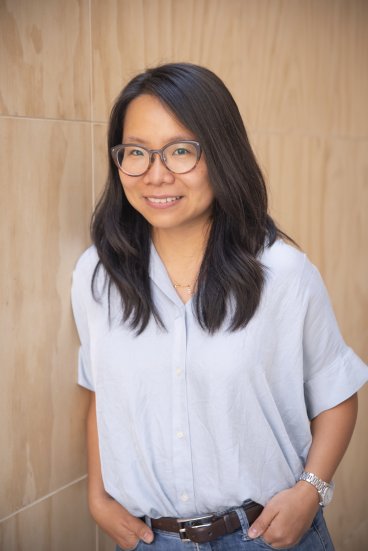
About: Xiaojing (Ruby) Fu is an Assistant Professor of Mechanical and Civil Engineering at California Institute of Technology. She received her BS in Applied Mathematics from Clarkson University, a MS in Computational Engineering, and a PhD in Civil & Environmental Engineering from MIT. She is awarded the Miller Postdoctoral Fellowship at UC Berkeley in 2018, hosted by the Earth & Planetary Science department. She joined Caltech in 2021 and leads the Mechanics of Porous Media Flow group. Her group studies the physics of multiphase fluid mechanics through porous media and how it shapes our natural and engineered environments, using theory, modeling, and laboratory experiments.